Human Neuroimaging Laboratory
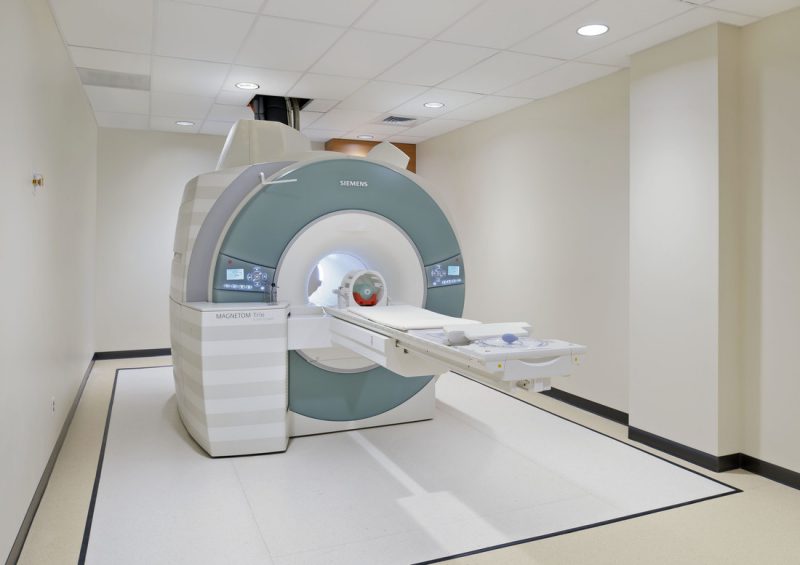
The Human Neuroimaging Laboratory's research projects span a wide variety of fields, including neuroscience, psychology, political science, law, and economics. The laboratory contains two research-dedicated Siemens functional magnetic resonance imaging devices in Roanoke, as well as one at our satellite location in Blacksburg; this allows for up to three research participants to be scanned simultaneously while participating in a negotiation or game in a novel technique called hyperscanning.
Faculty members throughout the institute have access to collaborate with the Human Neuroimaging Laboratory researchers and technicians. Faculty in the Fralin Biomedical Research Institute's Addiction Recovery Research Center, Center for Transformative Health Behaviors, and Computational Psychiatry Unit use the fMRI technology frequently to conduct human research studies.
fMRI stands for functional magnetic resonance imaging. In order to understand how it works, it is first necessary to understand conventional magnetic resonance imaging, or MRI. MRI is a technique for producing astonishingly detailed images of the brain or other bodily structures (see Fig 1). These images demonstrate the anatomy of the subject's brain at high resolution, and are used in virtually all modern hospitals to diagnose a wide variety of brain disorders, including brain tumors, multiple sclerosis, and stroke. MRI scanning uses a very strong magnet and radio waves to produce these spectacular images. The subject lies on a table, with his head surrounded by a large magnet. The magnet causes some of the atoms (or, more precisely, particles inside the atoms, called protons) inside the patient's head to align with the magnetic field. A pulse of radio waves is then directed at the patient's head and some of it is absorbed by the protons, knocking them out of alignment. The protons, however, gradually realign themselves, emitting radio waves as they do. These radio waves are captured by a radio receiver and are sent to a computer, which constructs the brain image. The patient cannot sense either the magnet or the radio waves; in fact, the patient only knows the machine is working because of the noise it makes during scanning. Different parts of the brain respond to the radio waves differently, and emit slightly different radio signals depending, among other things, on the local water and fat content. The computer that receives all of the signals, therefore, is able to distinguish one brain structure from another, and produce remarkable anatomical images, such as that shown in Figure 1.
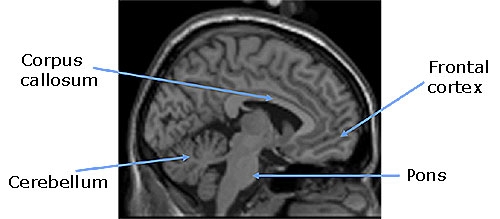
Functional MRI, or fMRI, was developed in the early 1990s and is a variation of magnetic resonance imaging. It uses a conventional MRI scanner, but takes advantage of two additional phenomena. The first is that blood contains iron, which is the oxygen-carrying part of hemoglobin inside red blood cells. Iron atoms cause small distortions in the magnetic field around them. Not all iron atoms do this: for the case of blood, only iron not bound to oxygen does this ("deoxyhemoglobin"). The second key phenomenon underlying fMRI is the physiological principle that whenever any part of the brain becomes active, the small blood vessels in that localized region dilate, causing more blood to rush in. The blood is presumably needed to provide extra oxygen and fuel (glucose) for the active brain cells. The result, however, is that a large amount of freshly oxygenated blood pours into any activated brain structure, reducing the amount of oxygen-free (deoxy) hemoglobin. This causes a small change in the magnetic field, and thus the MRI signal, in the active region. In the early 1990s, it was shown that an MRI scanner can be used to detect this small change in the signal, and thus detect which areas of the brain have been activated. So, for example, if a patient lying in a scanner is suddenly shown a flash of light, the visual cortex in his brain will become activated, blood flow there will quickly increase, and the MRI signal will change. The result is usually displayed as a patchy area of color, representing the brain area activated, superimposed upon a conventional, high-resolution, gray-scale image of the subject's brain (Fig 2). The signal is often called a BOLD signal, standing for Blood Oxygen Level Dependent signal.
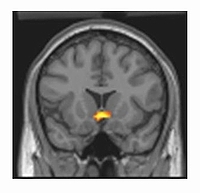
In summary, both conventional and functional MRI use a powerful magnet and radio waves to produce images of the brain. Conventional MRI images show beautifully detailed anatomy, and are an essential part of modern medicine. In fMRI, the same scanner is optimized to detect small changes in blood flow in the brain in response to scientifically designed stimuli. In principle, fMRI can be used to observe the activation of brain structures in response to almost any kind of brief stimulation, ranging from sounds, to visual images, to gentle touching of the skin. Currently, fMRI is being used across the world as a powerful neuroscientific research tool to study how the brain works, although some medical applications are being discovered as well.
The Roanoke Brain Study is part of a large-scale research endeavor based at the Fralin Biomedical Research Institute. Leading neuroscientists across a broad range of expertise are conducting research on multiple aspects of neurobiology, cognition, and behavior. The study combines novel approaches in functional brain imaging, computational psychiatry, and genetics to study features of decision behavior and how those behaviors change in people over the course of their lives. The aim is to create a global information resource for scientists, physicians, patients, and policy makers seeking insight into processes of decision-making to facilitate healthier lives.
The primary task will be an fMRI scan, a non-invasive imaging tool that allows us to capture images of the brain and its activity while volunteers view images, play games, or interact with other people. Volunteers may also be asked to provide a small sample of DNA, fill out questionnaires, play simple games on the computer, or interact with other people inside or outside the MRI scanner.
Highlights
- Target participation: 5,000 healthy subjects ages 18-85
- Project Headquarters: The Fralin Biomedical Research Institute at VTC in Roanoke, Virginia.
- Goal: To understand how genes and environment contribute to brain strategies for decision-making in all aspects of life.
- Approach: Longitudinal analysis of human behavior, its development, maintenance, and changes with aging
- Techniques: Study of decision-making, social interaction, and cognition using genomic analysis, behavioral analysis, and functional brain imaging
Further research (side projects)
- Visualising brain activities of academics during brainstorming (based on 100 academic experts involved in academic research services)
- Decision making under pressure research: group of students under deadlines (two days to complete writing assignment), with an opportunity to get a paper from a popular essay writing service but risk to get caught and be thrown away from university
- Studying creativity: brain activity while playing board games and music instruments (based on 100 student volunteers)
How to Get Involved
The first visit will take approximately 2-4 hours to complete. Subjects will visit the Human Neuroimaging Lab headquarters in Roanoke to register, determine eligibility, and participate in behavioral evaluation, fMRI scanning. We may collect a saliva sample for genetic analysis. If you are interested in learning more about the study or if you want to volunteer, visit our ongoing study page for more information.
Hyperscanning is a method by which multiple subjects, each in a separate MRI scanner, can interact with one another while their brains are simultaneously scanned. The hyperscanning technology permits the study of the brain responses that underlie important social interactions. The technique was developed by Read Montague of Virginia Tech with the Hyperscan software development taking place at Baylor College of Medicine under the direction of Dr. Montague during his previous appointment.
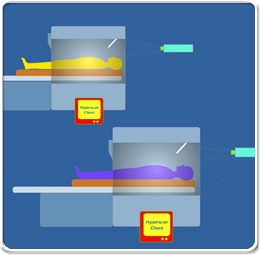
Hyperscanning technology allows, for the first time, a high resolution, simultaneous view of the functional neuroanatomy of two or more human brains engaged in a social interaction. The types of social interactions that can be studied are diverse, ranging from competitive games, to cooperative tasks, to musical performances. The only limitation is that the subjects must hold their head very still. All communication, at least currently, must be performed by movements of the wrists and fingers, usually using devices similar to a keyboard or a mouse. There are many medical research applications. Patients with diseases that predominantly affect their capacity for social interaction, such as autistic children, can be studied while they attempt to interact with normal subjects. Maternal neglect can be studied in terms of the brain activity in mother and child during an interaction.
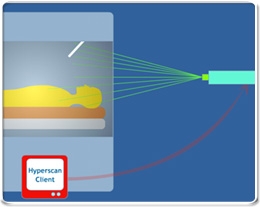
Hyperscanning software uses the Internet to allow one scientist to control multiple scanners, even if they are located thousands of miles apart in different centers. The scientist running the experiment controls the nature and the timing of the sensory stimuli that are delivered to the subjects. He or she also controls the initiation and termination of scanning in each of the scanners, and receives all of the data generated by the scanners. The subjects can react to the stimuli they receive by pressing on buttons or moving a joystick, and their responses can be relayed immediately to the other subjects. For example, two subjects could compete against each other in a digital video game, controlled remotely by an experimenter. Each time a move is made, the brain of the subject making the move is scanned, while the brain of the subject watching the move is simultaneously scanned.
Recent News from the Center for Human Neuroscience Research
-
Article ItemFellowship will help doctoral student explore the neuroscience behind yoga, meditation , article
Nishika Raheja wants to better understand the neurochemistry behind mindfulness practices. She will be supported in that work with a Ray A. Gaskins fellowship for students conducting research at the Fralin Biomedical Research Institute.
Date: Dec 12, 2024 -
Article Item'Curious Conversations' podcast: Read Montague talks about brain chemistry and neuroeconomics , article
Montague talks about the role of dopamine and serotonin in learning, motivation, memory, mood, and decision-making.
Date: Sep 23, 2024 -
Article ItemFralin Biomedical Research Institute at VTC and Virginia Tech shine as innovations receive TechNite acclaim , article
The Roanoke-Blacksburg Technology Council’s TechNite awards turned a spotlight on translational research taking place at Fralin Biomedical Research Institute at VTC and throughout the region.
Date: May 15, 2024 -
Article ItemMedical student plays important role in pioneering epilepsy research , article
Abhishek Bhutada worked with researchers at the Fralin Biomedical Research Institute at VTC to test a new type of equipment that proves to be a reliable guide for treatment options for people with drug-resistant epilepsy and is more comfortable and less invasive than traditional methods.
Date: Mar 07, 2024 -
Article Item11th annual Brain School digs into mysteries of the brain, emerging science behind weight-loss drugs , article
Researchers invite the community to the Fralin Biomedical Research Institute to share in their passion for science during an interactive brain awareness event, including presentations and panel discussion on “The Craving Brain: Food and Drugs.”
Date: Mar 01, 2024 -
Article ItemFirst-in-humans discovery reveals brain chemicals working to influence social behavior , article
For the first time, researchers show decisions made in a social context may spring from the dynamic interactions of dopamine and serotonin.
Date: Feb 26, 2024